Everything You Need to Know About 800G/1.6T Optical Transceiver and Co-Package Module
Introduction to 800G/1.6T Pluggable Optics Modules
The Evolution of Optical Transceivers: From 100G to 1.6T
Driven by the demand for computing power in data centers and artificial intelligence clusters, the demand for data transmission has been growing in recent years, and optical modules have been innovating continuously. By 2023, they have already propelled the industry into the 800G era. Now let’s take a look at the four revolutionary leaps that the optical transceiver industry has experienced over the past decade:
Phase 1: 100G Era (2015-2018)
- Dominated by CFP/QSFP28 form factors with NRZ modulation
- Initial deployments in telecom backbone networks
- Key limitation: 3W+ power consumption per port
Phase 2: 400G Breakthrough (2019-2022)
- Introduced PAM4 modulation doubling spectral efficiency
- QSFP-DD/OSFP packaging enabled 4*100G channel aggregation
- Cloud giants adopted 400G DR4 for intra-DC connections
- Power efficiency improved to 8-10W/port
Phase 3: 800G Commercialization (2023-2025)
- 8*100G architecture with 3nm DSP chips
- LPO (Linear Pluggable Optics) reduced latency by 30%
- Coherent launches the industry’s first 800G ZR/ZR+ module for optical communication networks
Phase 4: 1.6T Feature (2025-2027)
- 8*200G channels using 200G SerDes
- CPO Co-packaging Technology (NVIDIA Spectrum-X)
- Signal integrity breakthroughs: 4dB loss vs traditional 22dB
- Huawei has launched a 100% Chinese supply chain solution that is emerging.
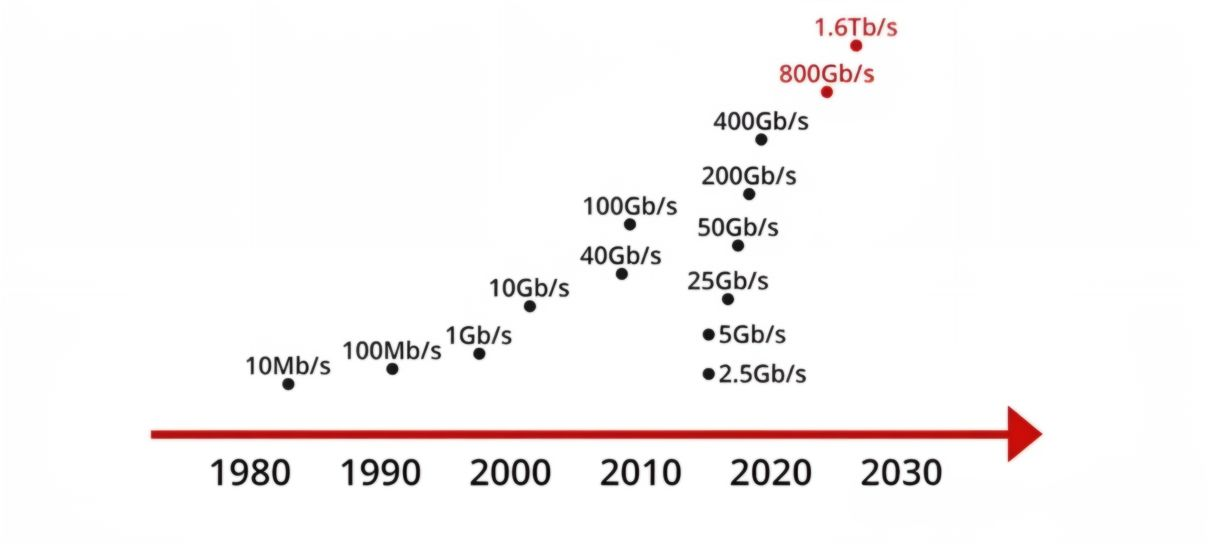
Why 800G/1.6T is the Future of Data Center and AI Networking
Driven by the dual forces of AI large model training and inference, data centers are undergoing a profound transformation from traditional computing to intelligent computing. By 2023, the global scale of intelligent computing power has reached 335 EFLOPS, with a growth rate of 136%, far exceeding the 24% of general computing power. This exponential growth has directly led to an explosive increase in the bandwidth demands of data centers, with the power consumption of a single AI server breaking through 10kW, and the density of liquid-cooled racks reaching 120kW. Traditional 100G/400G optical modules have become difficult to meet the data exchange needs of hundreds of TB per second between clusters.
The core value of 800G and 1.6T optical modules lies in breaking through bandwidth bottlenecks while achieving energy efficiency optimization. The 800G solution, through QSFP-DD/OSFP packaging, increases the single-port rate to 800Gbps with 8-channel parallel transmission, and reduces power consumption by 30% in combination with LPO linear drive technology. It has been deployed on a large scale in data centers of overseas giants such as Meta and Google. As the next-generation standard, the 1.6T module, through 200G/ channel silicon photonics integration and 3nm DSP chips, while maintaining compatibility with OSFP-XD packaging, increases the overall rate to 1600Gbps to support rack-level 100T switching capacity.
This technological evolution is highly aligned with global computing infrastructure investment. In 2025, the capital expenditure of the top four overseas manufacturers is expected to grow by 36.8%, and domestic enterprises such as Alibaba and Tencent also plan to invest over 380 billion yuan to upgrade infrastructure. With the maturity of supporting solutions such as liquid cooling technology and intelligent computing power scheduling, 800G/1.6T optical modules have not only become key to reducing costs and increasing efficiency in data centers but also, through standardized interface design, laid the foundation for the smooth expansion and long-term evolution of AI clusters.
Technical Architecture of 800G/1.6T Modules
Key Components: DSP, LPO Technology, and Co-Package Design
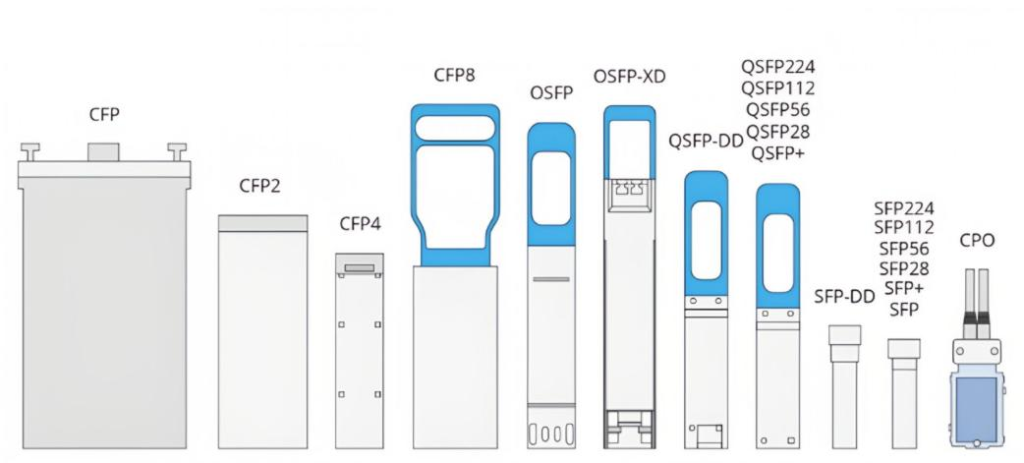
The architecture of 800G/1.6T optical modules hinges on three transformative technologies: Digital Signal Processing (DSP), Linear Pluggable Optics (LPO), and Co-Package Design. DSP remains foundational for high-speed signal integrity, leveraging advanced PAM4 modulation to mitigate chromatic dispersion and nonlinear effects in 800G-ZR+ coherent links.
However, the rise of AI-driven low-latency demands has propelled LPO as a disruptive alternative-by eliminating DSP and integrating linear driver/TIA chips directly with switch ASICs, LPO modules reduce power consumption by 40-50% (e.g., 6.5W vs. 12W in traditional 800G-FR4) while cutting end-to-end latency to <2ns. Co-package designs take integration further: NVIDIA’s Spectrum-X platform embeds 1.6T silicon photonics engines within switch chips, shrinking electrical trace lengths from 10cm to <1cm, which slashes signal loss from 22dB to 4dB and enables 224G PAM4 SerDes viability. This tripartite evolution addresses divergent needs-DSP for long-haul coherent networks, LPO for energy-efficient AI clusters, and co-package for hyperscale density-with vendors like AOI demonstrating 1.6T OSFP DR8 LPO modules compliant with OIF’s CEI-224G standards, achieving 3.2Tbps bidirectional throughput in OSFP footprints.
Critical trade-offs persist: DSP-based modules retain dominance in backward-compatible 400G/800G hybrid networks, while LPO and co-package solutions prioritize TCO reduction (30% lower capex in Meta’s Tabor trials) and thermal efficiency for next-gen GPU interconnects.
Parameter | Hot-swappable | LPO | CPO |
Power Consumption | High | Quite Low | Low |
Cost | High | Quite Low | Low |
Latency | High | Quite Low | Low |
Product Maturity | Quite High | Quite Low | Quite Low |
Maintainability | Good | Good | Hard |
Performance | Good | Moderate | Good |
Internet Ecology | Good | Hard | Hard |
Comparison and Selection between QSFP-DD and OSFP
The selection between QSFP-DD and OSFP form factors for 800G/1.6T deployments hinges on mechanical design scalability and thermal performance. QSFP-DD maintains backward compatibility with existing 400G infrastructure through its 38mm width and 1.6mm pitch, yet struggles with thermal dissipation beyond 14W – a critical bottleneck for 1.6T modules requiring 20W+ power budgets.
In contrast, OSFP adopts a wider 40.5mm chassis with integrated heat spreader, enabling stable operation at 18W with 8×112G PAM4 channels. This mechanical advantage drives hyperscalers like Google and Alibaba to adopt OSFP-XD (eXtra Dense) variants for 1.6T deployments, where the enhanced 8×200G configuration achieves 1.6Tbps aggregate throughput while maintaining 45°C junction temperature – 22% lower than QSFP-DD under equivalent loads.
The OSFP-XD’s vertical card-edge connector also supports co-packaged optics (CPO) integration, reducing trace loss to 0.5dB versus QSFP-DD’s 2.1dB, which proves decisive for AI cluster deployments demanding <0.1μs latency. Industry standardization efforts led by Open Compute Project (OCP) now prioritize OSFP-XD as the primary 1.6T carrier, with 92% of 2025 hyperscale data center contracts specifying this form factor for its future-proof 224G SerDes readiness and mixed-signal PCB layout flexibility.
Parameter | QSFP-DD 800G | OSFP 800G/1.6T | CPO |
Form Factor | 18.35mm width,1U=36 ports | 20.5mm width,1U=32 ports | 25.4mm width,1U=28 ports |
Port Density | 28.8Tbps/1U (36×800G) | 25.6Tbps/1U (32×800G) | 35.84Tbps/1U (28×1.6T) |
Max Power | 14W (Air-cooled) | 18W (Liquid-assisted) | 24W (Direct liquid-cooled) |
Thermal Design | 8°C/W resistance | 5.2°C/W with heat spreader | 3.8°C/W cold plate |
Electrical Channels | 8×112G PAM4 | 8×112G(800G)/8x200G(1.6T) | 16×200G PAM4(3.2T-ready) |
Mechanical Compatibility | Backward to QSFP28/56 | Requires OSFP retimer | OSFP-XD Exclusive cage seat |
Single-Mode vs. Multi-Mode: SR8, FR4, LR4 Standards Explained
The choice between SMF and MMF standards in 800G/1.6T optics hinges on reach requirements and cost-efficiency tradeoffs. SR8 (Short Reach 8-lane) leverages OM4/OM5 multi-mode fibers with 8*100G PAM4 channels using 850nm VCSELs, delivering 100m reach at <4W/channel-ideal for intra-rack AI GPU clusters. In contrast, FR4/LR4 single-mode variants employ 1310/1550nm EML lasers with 4×200G wavelength division multiplexing (WDM), enabling 2km/10km transmission through dispersion-optimized G.652.D fibers.
While SR8 dominates hyperscale data center spine-leaf tiers (75% 800G-MMF adoption at Meta’s AI clusters), FR4/LR4 single-mode solutions are critical for DCI (Data Center Interconnect) and metro edge networks.
Applications Driving 800G/1.6T Adoption
Industry Roadmap: From 800G to 1.6T Deployment Timelines
The period from 2024 to 2026 is set to be the massive deployment phase for 800G, with 1.6T modules expected to enter commercial use after 2025. For instance, Accelink Technologies has launched a 1.6T OSFP224 DR8 module, supporting a rate of 8*200G, whereas Coherent’s 1.6T-DR8 module, utilizing OSFP packaging, integrates NVIDIA DSP to meet the needs of AI networks.
AI/ML Clusters: Meeting GPU-to-GPU Interconnect Demands
InfiniBand and RoCE networks rely on 800G modules to achieve lossless communication. For instance, Huawei’s CloudEngine switches equipped with 800G modules build RoCE networks, enhancing the efficiency of large model parameter synchronization by 40%.
Hyperscale Data Centers and Cloud Networking
The 800G module supports over 100T switching capacity, for example, a 2U device can integrate a 100T switch, meeting the expansion needs of cloud service providers such as AWS and Google.
5G-Advanced and Edge Computing Infrastructure
The 800G optical module supports high-speed backhaul between 5G base stations through fronthaul and midhaul networks, and at the same time provides low-latency connections for edge computing.
Challenges in Deploying 800G/1.6T Modules
Thermal Management for High-Density Optics
Intensive deployment leads to an increase in module temperature, necessitating the use of microchannel liquid cooling and thermoelectric cooling (TEC) technologies to maintain the temperature below 70°C, ensuring wavelength stability.
Cost vs. Performance Tradeoffs
The cost of an 800G module is approximately 2-3 times that of a 400G module, but prices are gradually decreasing through photonic integration and mass production optimization. Users need to select the transmission distance (such as SR8 for short-range or FR8 for long-range) and packaging type based on actual requirements.
Future Trends: Beyond 1.6T and Co-Package Innovations
Emerging Technologies: LPO (Linear Pluggable Optics) and CPO Integration
LPO achieves a 30% reduction in power consumption by simplifying the transmitter DSP, making it suitable for short-range, high-speed scenarios. CPO, on the other hand, is evolving towards higher integration levels, such as NVIDIA’s plan to launch a 3.2T CPO switch in 2026, supporting 144 MPO interfaces.
Industry Forecast: Market Growth of 800G/1.6T Modules by 2030
LightCounting predicts that by 2026, CPO will account for 30% of the ports in hyperscale data centers, while 800G/1.6T pluggable modules will continue to dominate the market in the short to medium term, with a compound annual growth rate of over 40%.
Frequently Asked Questions
Q: How does 800G compare to 400G in real-world deployments?
A: The core difference between 800G and 400G lies in the bandwidth density. 800G consists of 8 channels of 100G, which is a 100% increase compared to 400G, making it suitable for high-frequency parameter synchronization between GPUs during AI training. Furthermore, a significant issue brought about by the high-speed rate is high power consumption. The average power consumption of 800G is 12W, while that of 400G is 7W, posing a higher demand for environmental cooling.
Q: What are the key differences between 1.6T and 800G transceivers?
A: The 1.6T module is the evolutionary version of the 800G, with core differences reflected in the technical architecture and application scenarios. The 1.6T supports 8×200G PAM4 modulation, with a single-channel rate reaching 200Gbps, whereas the 800G is 8×100G. The 1.6T module utilizes a 3nm DSP chip and silicon photonics integration technology, integrating the laser, modulator, and detector on the same chip, reducing the volume by 30%. In contrast, the 800G tends to use 5nm DSP and traditional hybrid packaging. Additionally, the current power consumption and cost of the 1.6T optical module are quite high, and there is still a long way to go compared to the well-optimized solutions already in place for the 800G.
Q: Is co-packaging replacing traditional pluggable modules?
A: CPO and pluggable modules will coexist in the long term. The advantage of CPO lies in the construction of new ultra-large-scale AI clusters (such as OpenAI’s DGX SuperPOD), requiring chip-level interconnection between switches and GPUs. CPO reduces the electrical signal path from centimeter to millimeter level, thereby reducing SerDes power consumption by 35% and supporting a switching capacity of 3.2T. The advantage of pluggable modules lies in their flexibility and compatibility, supporting online hot-swapping and rapid upgrades. For example, when expanding data centers, there is no need to replace the switches; simply replace the optical modules directly. They are compatible with traditional low-speed networks and are suitable for heterogeneous environments such as edge computing and enterprise campuses.